GENE EDITING
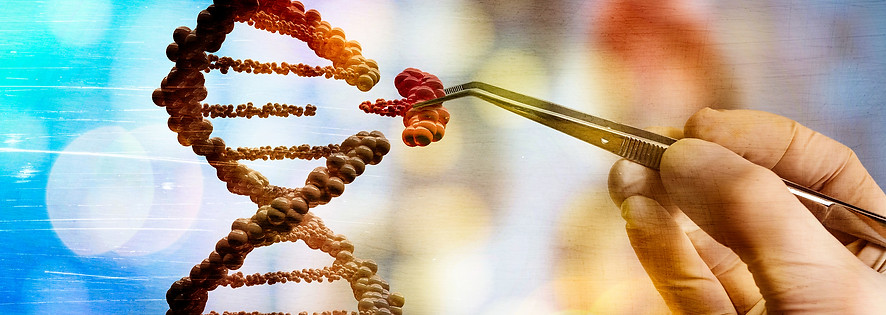
Introduction to Gene Editing:
​
Gene editing is a groundbreaking scientific technique that enables precise modifications to an organism's DNA. This revolutionary approach leverages advanced technologies, such as CRISPR-Cas9, TALENs, and zinc-finger nucleases, to target specific genes for alteration. These tools act like molecular scissors, cutting DNA at designated locations to add, remove, or modify genetic material. One of the most widely used methods, CRISPR-Cas9, has transformed gene editing due to its efficiency, accuracy, and ease of use. Originating from a natural defense mechanism in bacteria, CRISPR uses a guide RNA to pinpoint a specific DNA sequence and a Cas9 enzyme to make the cut. Once the DNA is edited, the cell's natural repair mechanisms either fix the gene or incorporate a new segment of DNA. Applications of gene editing span across various fields. In medicine, it holds promise for curing genetic disorders like cystic fibrosis and sickle cell anemia. In agriculture, it enables the creation of pest-resistant crops and enhanced food yields. Additionally, gene editing offers innovative solutions for addressing global challenges, such as improving environmental sustainability and combating infectious diseases.
​
Purpose of Gene Editing in Medicine:
The primary goal of gene editing in medicine is to correct genetic mutations responsible for diseases, enhance therapeutic strategies, and develop innovative treatments. Key medical applications include:
-
Treating Genetic Disorders: Correcting mutations in diseases such as cystic fibrosis, sickle cell anemia, and muscular dystrophy.
-
Cancer Therapy: Engineering immune cells, like T-cells, to target and destroy cancer cells.
-
Infectious Disease Control: Modifying the genetic material of pathogens or vectors, such as mosquitoes, to curb diseases like malaria.
​
Early Stages of Gene Editing Development:
​
-
The development of gene editing began with the discovery of restriction enzymes in the 1970s, marking the first tools for cutting DNA at specific sites. This breakthrough enabled scientists to manipulate genetic material, laying the foundation for recombinant DNA technology.
-
In the 1990s, zinc-finger nucleases (ZFNs) emerged as the first customizable gene-editing tools, offering greater precision in targeting specific DNA sequences. Shortly after, transcription activator-like effector nucleases (TALENs) were developed, providing a more versatile and accessible approach to DNA editing.
-
The field truly advanced in 2012 with the discovery and adaptation of CRISPR-Cas9, a bacterial defense mechanism repurposed for gene editing. Its simplicity, accuracy, and efficiency revolutionized genetic research, making it widely accessible to scientists across disciplines.
-
These early innovations paved the way for modern gene editing, enabling breakthroughs in medicine, agriculture, and biotechnology, while sparking ethical debates about its applications and implications.
Methodology and Tools in Gene Editing:
​
Gene editing involves precise modifications to an organism’s DNA, achieved through advanced tools and methodologies. The process generally includes three key steps: target identification, DNA cleavage, and repair or modification of the genetic material.
Target Identification: Scientists first identify the specific DNA sequence to be edited. This involves using bioinformatics tools to analyze and design precise genetic targets.
DNA Cleavage: Special enzymes, known as nucleases, are used to cut the DNA at the desired location. The most prominent tools for this step include:
-
CRISPR-Cas9: A highly efficient system where the Cas9 protein, guided by RNA, cuts the DNA at the targeted sequence.
-
TALENs: Proteins engineered to bind specific DNA sequences and induce cuts.
-
Zinc-Finger Nucleases (ZFNs): Complex proteins designed for specific DNA interactions.
DNA Repair and Modification: Once the DNA is cut, the cell’s natural repair mechanisms are activated:
-
Non-Homologous End Joining (NHEJ) introduces small mutations to disable genes.
-
Homology-Directed Repair (HDR) allows for precise insertion or correction using a provided DNA template.
These tools and methodologies underpin modern advances in gene editing, driving innovations in fields like medicine, agriculture, and environmental science.
​
New Innovations in Gene Editing:
​
Gene editing is rapidly advancing, with researchers continually pushing the boundaries of what is possible. Some of the most exciting recent innovations include:
Base Editing:
-
A cutting-edge technology that allows precise changes to individual DNA bases (e.g., A→G or C→T) without cutting the DNA strands.
-
Significantly reduces the risk of unintended genetic alterations and is particularly useful for treating single-gene disorders such as sickle cell anemia.
Prime Editing:
-
Dubbed the "word processor" of gene editing, this tool enables scientists to insert, delete, or replace DNA sequences with remarkable precision.
-
Unlike CRISPR-Cas9, prime editing avoids creating double-strand breaks, reducing the risk of errors and offering broader applications for complex genetic corrections.​
CRISPR-Cas Variants:
-
Development of new CRISPR systems (e.g., Cas12, Cas13) tailored for specific tasks such as targeting RNA or DNA with greater efficiency.
-
RNA-targeting CRISPR tools are particularly promising for treating conditions like Huntington’s disease and certain viral infections.
Gene Drives:
-
An innovative application of CRISPR to spread genetic traits through populations of organisms.
-
For example, gene drives are being used to combat malaria by modifying mosquito populations to reduce their ability to transmit the disease.
Epigenome Editing:
-
Focuses on modifying the chemical markers that regulate gene expression without altering the underlying DNA sequence.
-
Promises applications in reprogramming cells for regenerative medicine or treating conditions like diabetes and cancer.
-
These innovations are expanding the possibilities of gene editing, bringing us closer to transformative solutions for healthcare, agriculture, and environmental challenges.
​
Applications of Gene Editing:
​
Gene editing has revolutionized various fields by enabling precise modifications to genetic material. Its versatility and efficiency have paved the way for numerous applications:
Medicine:
Treatment of Genetic Disorders: Gene editing offers potential cures for diseases like cystic fibrosis, sickle cell anemia, and Huntington’s disease by correcting defective genes.
Cancer Therapy: CRISPR is used to modify immune cells, enhancing their ability to target and destroy cancer cells.
Infectious Diseases: Gene editing shows promise in combating viral infections like HIV by altering host DNA or directly targeting the virus.
Agriculture:
Improved Crops: Editing plant genomes can enhance resistance to pests, diseases, and environmental stress, while improving yield and nutritional value.
Livestock Improvement: Gene editing helps in disease resistance and improving desirable traits in animals.
Environmental Applications:
Conservation: It aids in protecting endangered species by addressing genetic vulnerabilities.
Bioremediation: Genetically engineered microbes can break down pollutants and clean up the environment.
Industrial Biotechnology:
Biofuel Production: Edited microorganisms enhance the production of sustainable biofuels.
Synthetic Biology: Gene editing supports the creation of novel materials and chemicals.
These applications showcase the transformative potential of gene editing ivn addressing global challenges.
​
Pros and Cons of Gene Editing:
Gene editing holds immense potential, but it also raises ethical, technical, and societal concerns. Here’s a balanced overview of its
Pros:
Medical Advancements:
· Offers potential cures for genetic disorders like sickle cell anemia, cystic fibrosis, and muscular dystrophy.
· Enhances cancer immunotherapy and treatments for infectious diseases like HIV.
Agricultural Benefits:
· Improves crop yield, pest resistance, and resilience to environmental changes.
· Enhances nutritional content of food, addressing malnutrition.
Environmental Impact:
· Supports conservation efforts by protecting endangered species.
· Enables bioremediation, using edited organisms to clean pollutants.
Economic Growth:
· Drives innovation in biotechnology, fostering job creation and industry growth.
Cons:
Ethical Concerns:
· Raises questions about editing human embryos and "designer babies."
· Challenges societal views on altering natural processes.
Unintended Effects
· Potential off-target mutations could lead to unintended consequences.
· Long-term effects on ecosystems and organisms are still uncertain.
Accessibility and Equity
· Advanced technologies may not be equally accessible, widening socioeconomic gaps.
· Risks of misuse for non-ethical purposes, such as biological weapons.
Regulatory Challenges
· Lack of universal guidelines creates ambiguity in its safe and ethical use.
Balancing these aspects is vital to harness the benefits of gene editing responsibly while addressing its challenges.
​
Prescribed Book:​
​
E-book URL: CRISPR-Based Gene Editing